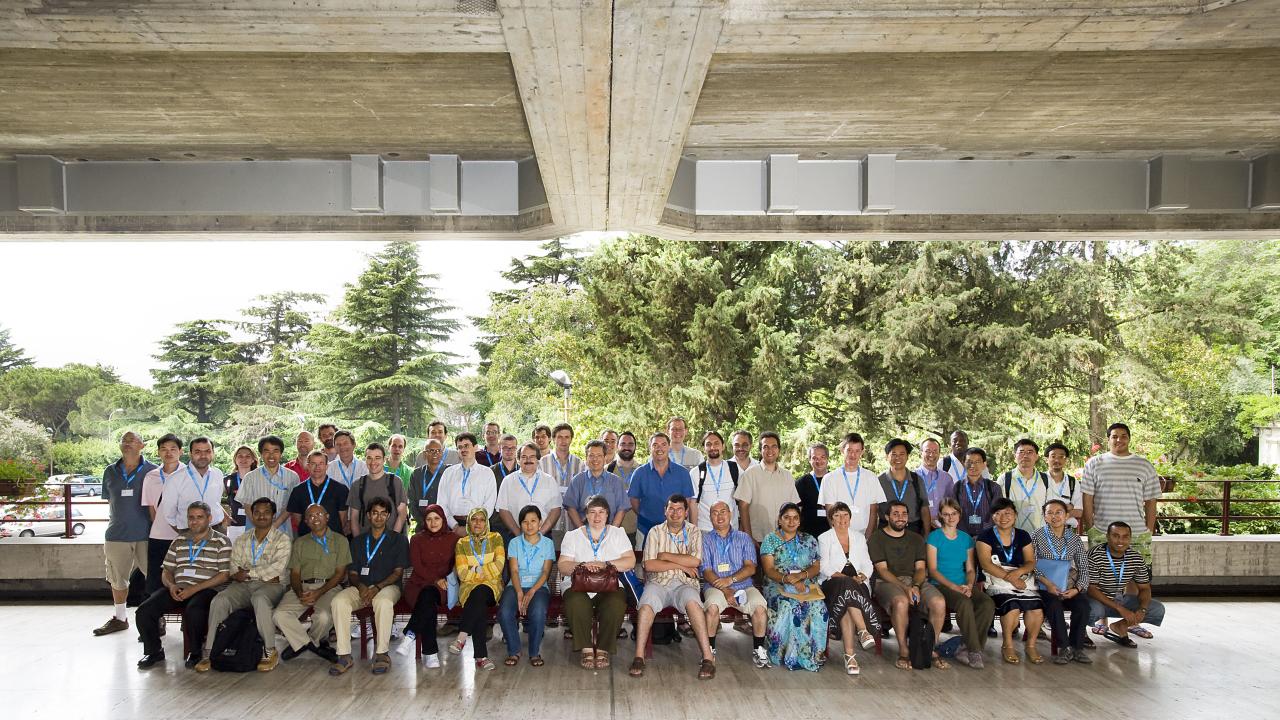
Some materials are so unsettled on the quantum level that they behave unusually in the classical world. In July, 82 researchers attended ICTP's Workshop on Emergence of New States of Matter in Magnetic Systems and Beyond to discuss such strange behaviour: new states of matter that arise in frustrated magnets and strongly correlated systems. Studying these forms of matter could help unlock the mystery of high-temperature superconductivity.
These states derive from complicated interactions between many particles and the geometry of the underlying crystal lattices. Such collective phenomena have fascinated physicists for quite some time, says participant Alexei Tsvelik, a condensed matter theorist at Brookhaven National Laboratory in the United States. But interest in the topic received a huge boost from the discovery of high temperature superconductors in 1987. It promised technological advances such as hovering trains and super-efficient wires. Twenty-three years later, researchers have still not found cheap materials that dependably superconduct above the boiling point of liquid nitrogen (77 K). And there is no established explanation of exactly how high-temperature superconductivity works.
According to a school of thought initiated by the Nobel physicist P. W. Anderson, the seeds of high-temperature superconductivity can be found even in the insulating state of the given materials. Only a slight change in the composition of an insulator can transform it into a high-temperature superconductor.
Broadly speaking, some insulators cause electrons to act as though they attract each other. Electrons with opposing spins become paired. When a few of the electrons are removed, or “holes” are introduced, an electric current has room to flow smoothly. But when the current disrupts the spin order, it experiences a sort of resistance.
"Qualitatively, you can imagine that the motion of these holes would be assisted if the underlying magnetic state were disordered, like a liquid," said Brijesh Kumar, a junior ICTP associate and an organizer of the workshop.
Enter the theoretically predicted spin liquid: a crystal in which electron spins remain disordered and fluctuate even at absolute zero.
“The spin liquid has been something like a philosopher's stone in superconductivity,” said Tsvelik, who presented his work on coupled spin chains.
Spin liquids are thought to arise in complex crystals that include copper, vanadium, or other transition metals. In many of these compounds, there is always one unpaired electron for any two that are paired. The spins are predicted to fluctuate constantly.
Workshop participants have been probing promising compounds experimentally and theoretically, and in some cases, emulating them using cold atoms on optical lattices.
"People at this conference showed some experimental situations where even at a few millikelvins, they haven’t seen any spin order," Tsvelik said.
The basis of superconductivity—that electrons in solids appear to attract each other—fits well with the broader philosophical basis of the workshop, Tsvelik added. Physicists often study elementary particles to understand complex behaviour. Participants at this workshop take an opposite approach.
"The philosophical idea here is that some things are difficult or impossible to envisage when you think of single particles," he said. "Looking at a water molecule, would you imagine that there are waves on the sea?"